A microphone (also mic or mike) is a transducer that converts sound waves into electrical signals.
In its most basic form, a microphone consists of a diaphragm, a magnet, and a coil. When sound waves hit the diaphragm, it vibrates, moving the coil through the magnetic field and generating an electrical current. This current is the audio signal that gets sent to speakers, recording devices, or other electronics.
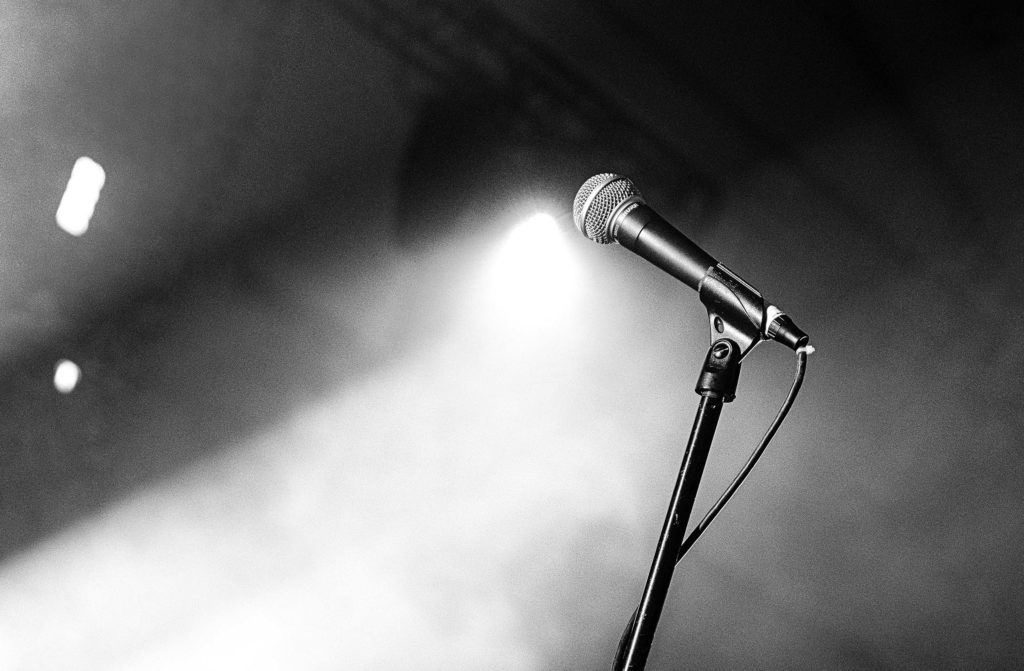
What are Microphones: Table of Contents
Microphones allow us to capture and manipulate sound in ways that have revolutionized how we communicate. From telephony to music recording, radio broadcasting to hearing aids, microphones have become integral to technologies that define modern life.
While the basic operating principles have remained unchanged, microphone design has evolved enormously over the past century. Electromagnetic induction mics using coils and magnets gave way to capacitive condenser mics, then electret condenser mics, and most recently, MEMS microphones. Directional polar patterns emerged, essential for filtering noise and isolating sources. Wireless and digital transmission removed cables. Waterproofing enabled new applications. Materials science sharpened sensitivity and robustness.
Today’s microphones convert the faintest whispers into bytes of data. Multi-mic arrays focus recordings. Smart algorithms extract voices from crowded rooms. The human voice, once ephemeral, now streams without limit across time and space thanks to the microphone’s continual transformation.
This guide will cover everything from the physics of sound to the latest high-tech microphone advances. You’ll learn how these devices evolved from crude nineteenth century prototypes into the omnipresent, ultra-precise tools we know today. Whether you’re a musician, sound engineer, student, or simply microphone-curious, exploring the technology behind these clever gadgets will change how you hear the world. Let’s start by examining exactly how microphones work their auditory magic.
How Microphones Work
Understanding how microphones convert sound waves into electrical signals starts with the basic components common to most mics: a diaphragm, a magnet and a coil. The diaphragm is a thin, flexible membrane or surface that vibrates when it is struck by sound waves. In microphones, the diaphragm is often made of lightweight plastic, such as Mylar. Attached to the diaphragm is a coil of wire that sits within a fixed magnet. This coil is free to move up and down as the diaphragm vibrates.
How Sound Waves Are Converted Into Electrical Signals
When someone speaks, sings, or makes any noise near a microphone, they create vibrations known as sound waves. These waves ripple outwards through the air until they reach the microphone’s diaphragm.
The sound waves exert a force that pushes and pulls on the diaphragm, causing it to vibrate back and forth. This motion is nearly identical to the original pressure variations in the sound wave.
As the diaphragm moves inward and outward, it brings the attached coil along with it. With each vibration cycle, the coil moves back and forth through the magnetic field.
This is where the magic happens. According to the laws of electromagnetic induction, when a wire cuts through magnetic field lines, a current is generated in the wire.
So as the coil vibrates, it is constantly inducing current flows by slicing through the microphone’s steady magnetic field. The strength and direction of these currents change according to the diaphragm’s movement.
In this way, the pattern of the diaphragm’s vibrations—which match the original sound wave—get translated into an analogous pattern of electrical current. This alternating current is the electrical representation of the sound wave that can then be amplified, recorded, or transmitted.
Converting pressure waves into voltage or current is how all microphones bridge the divide between the mechanical world of sound and the electronic world of signals and circuits. The same fundamental principles govern the operation of a 19th century carbon mic and the micarrays in our smartphones. This clever electromechanical translation is what empowers microphones to capture and control sound.
The Main Microphone Components: Diaphragm, Magnet, and Coil
While microphones may look quite different on the outside, most contain the same essential internal components:
The Diaphragm
This thin, flexible membrane is attached to or contains the coil and moves along with it. The diaphragm material and design affect the microphone’s frequency response and sensitivity.
- Common materials include plastic, Mylar, metal, or specialized synthetic compounds.
- Size ranges from large 1.5 inch capsules to tiny MEMS diaphragms less than 1 mm wide.
- Shape can be circular, rectangular, or elliptical depending on pickup pattern.
The Magnet
Permanent bar magnets made of ferrous materials like iron or cobalt provide the static magnetic field.
- Stronger magnets improve microphone sensitivity but make damping difficult.
- Larger magnets increase sensitivity but also increase size and weight.
- Ring-shaped magnets are common in small microphones.
The Coil
This narrow loop of thin wire is attached to the diaphragm and positioned within the magnetic field.
- Coil width and position impact current induction and impedance.
- Coils typically have hundreds of turns of fine copper wire.
- Perfect coil alignment is critical for optimal performance.
While the electronics and housings differ vastly between mic models, this core electromagnetic system remains the key to any dynamic or condenser microphone’s operation. The genius of microphone pioneers like Emile Berliner was finding these simple components that could unlock sound.
Types of Microphones
Microphones come in a variety of shapes, sizes, and designs thanks to the many innovative ways engineers have devised to translate sound waves into electrical signals. Let’s explore some of the most common modern microphone types and how they work.
Dynamic Microphones
Dynamic microphones are the workhorses of live audio. They use a simple but effective electromagnetic design that has remained much the same since microphones first emerged in the late 1800s.
Also known as moving coil or induction mics, dynamics operate by moving an aluminum coil through a magnetic field. Attached to the coil is a lightweight, typically plastic diaphragm that vibrates when hit with sound waves.
As the coil moves up and down with the diaphragm, it pushes and pulls against magnetic lines of flux from a permanent magnet. This induces an AC current that matches the original soundwave.
Rugged and affordable, dynamic mics handle loud volumes and abuse. Their mid-boosted frequency response makes voices punch through mixes. Limited low and high end pickup reduces ambient noise.
Handheld vocal dynamics like the legendary Shure SM58 are a rock and pop mainstay. Their durability also suits them for miking guitar amps and drum kits. Even smartphones integrate tiny dynamic mics for calls.
However, for pristine studio recordings, condenser and ribbon mics are more in demand today. Dynamics’ lower output and sensitivity require more gain for the same volume level.
Still, for amplifying speech and vocals with articulation and clarity, the dynamic mic’s simple design has proven hard to beat. They make sound reinforcement and recording possible in the noisy real world.
Condenser Microphones
Condenser microphones use an electrically charged diaphragm and backplate to form a capacitor. Incoming sound waves move the diaphragm, changing the distance between it and the backplate, and thus the capacitance.
First patented by Wente and Thuras in 1916, condensers capture subtler nuances than dynamics. This makes them a top studio choice for vocals, strings, piano and other detailed recording.
Condensers require external power to charge the capacitor plates. Traditional “polarized” condensers use high voltage phantom power, while electret types have a permanently charged backplate and use standard mic power.
Their ultra lightweight diaphragms boast extended frequency response. Detailed transient response makes condensers a favorite for drum overheads. Pristine high end picks up breath tones and room ambience.
Supercardioid and shotgun models reject off-axis sounds, allowing more gain before feedback in live sound. Multi-pattern versatility aids flexible studio miking.
From vintage gems like the U47 to affordable large diaphragm models, condensers lend recordings transparency, richness, and accuracy. Their rise usurped dynamics in studio use and propelled microphone evolution.
Ribbon Microphones
Ribbon mics are dynamic microphones that use an ultra-thin corrugated aluminum ribbon suspended in a magnetic field instead of a coil. When sound waves vibrate the ribbon, it generates current through electromagnetic induction.
First developed in the 1920s, ribbon designs enjoyed early use in broadcast and recording before condensers overtook them. However, ribbon mics have seen a major comeback among modern musicians and engineers.
Ribbons offer a uniquely smooth, natural sound. Their bidirectional polar pattern provides strong off-axis rejection, mitigating room reflections. Delicate transient response excels on drums and guitars.
However, the ribbon element is fragile and easily damaged by plosives or high SPLs. Low output requires pre-amplification. Proximity effect boosts bass at close range.
Despite their fragility, ribbons withstand moisture and temperature extremes that disable condensers. This makes them a top choice for outdoor recording and live miking of guitar amps and brass.
From jazz horns, to bluegrass strings, to heavy rock wails, ribbons lend a silky, realistic texture. Their figure-8 directivity controls bleed between instruments. Vintage warmth meets precision presence.
Ribbons may lack refinement of condensers, but their natural uncolored sound makes voices and instruments shine. Second order harmonics add richness minus harsh digital edginess. That velvet fluidity is their allure.
Carbon Microphones
The first microphone able to accurately transmit speech was the carbon microphone, developed independently by David Edward Hughes and Emile Berliner in the late 1870s.
Carbon mics used loosely packed carbon granules between two metal plates. Voice vibrations caused varying pressure on the granules, which changed electrical resistance between the plates. This created an analogue of the sound wave.
Though primitive by today’s standards, carbon mics were a revolution in clear telephony and radio voice transmission. Their robustness made them standard for over half a century.
However, carbon mics had severe limitations in fidelity and frequency response. Output was noisy compared to condensers and dynamics. Their restricted frequency range and distortion made them unsuitable for music recording.
Bell Labs developed the first condenser mics in 1916 to overcome carbon mics’ deficiencies. As electronics advanced, carbon mics were rendered relics. Though incredibly historically important, they have little relevance to modern audio work.
Yet it’s astounding that granules of carbon could capture nuances of speech at all. The fundamental acoustic-to-electric principles pioneered in these rudimentary devices still enable crystal clear modern telecommunication and voice control.
Piezoelectric Microphones
Piezoelectric microphones use the phenomenon of piezoelectricity to directly convert mechanical vibrations into an electrical signal. They contain a piezoelectric material, usually a crystal or ceramic, coupled to a diaphragm.
When the diaphragm vibrates from sound waves, it strains the piezoelectric element. This deformation of the material’s crystal structure generates an AC voltage across the element.
Though less common than dynamics and condensers, piezoelectric mics offer unique advantages:
- Ultra wide frequency response up to 120 kHz for scientific measurement
- High sensitivity for quiet sounds down to 10 dB SPL
- Resistance to mechanical shock, temperature, and moisture
- Low cost compared to condensers
However, their high impedance output requires a preamp, and sensitivity varies widely between piezo materials. Cheap piezos suffer from distortion and microphonics. Quality has a price.
Applications include measurement mics, hydrophones, and contact mics for amplifying vibrations in solid objects. High Z piezo mics serve folk instruments like accordions and banjos with passive pickups.
For airy studio vocals, handhelds, and precision recording, piezos lack the finesse of condensers and dynamics. But for experiential mic needs from underwater to rocket launches, piezoelectricity gets sound without distortion.
Microphone Polar Patterns
The polar pattern or pickup pattern determines how sensitive a microphone is to sounds arriving from different directions. The pattern is often visualized as a polar diagram with concentric circles representing decibels of attenuation.
Understanding polar patterns allows choosing the right mic for an application and optimal positioning. Let’s examine the properties of the most common polar patterns.
Omnidirectional Microphones
Omnidirectional, or omni, mics pick up sound from all directions equally. Their polar pattern is a sphere, providing 360 degrees of equal sensitivity.
Sound can enter the mic capsule from any angle. This makes omnis excellent at capturing ambience and room tone. But it also means they lack directional selectivity and noise rejection.
Piano mics are often omnis to encompass the instrument’s broad soundboard. “Room mics” placed far from drum kits use omnis to give a spacious, natural sound with lots of reverberation.
Lavalier mics for television and theater are omnidirectional so sudden head turns don’t lose sound. Choir mics several feet back encompass the entire ensemble.
In stereo recording, omni mics prevent phase issues like comb filtering. Spaced omnis form a “stereo image” through level and arrival time differences.
However, omnis suffer from feedback when amplified in close miking applications. Their sensitivity to stage noise and monitors makes them a poor choice for vocals or spot miking.
If isolation isn’t required, omnis provide an open, bright, “natural” sound. But where directional control is needed, other patterns like cardioid and supercardioid are preferred.
Cardioid Microphones
The most common microphone polar pattern, cardioid mics pick up sound mainly from the front, with some pickup from the sides and minimal sound from the rear.
Their heart-shaped polar pattern provides directional selectivity, rejecting off-axis sound. This improves gain before feedback and isolation for close miking vocals, drums, guitar amps, and more.
However, cardioids exhibit proximity effect – increased bass response up close. They also color off-axis sounds due to their directional nature.
Cardioids strike a versatile balance between isolation and natural sound for studio recording and live sound. Their pronounced front response suits them for spot miking instruments, amplifiers, and vocalists.
Handheld vocal mics use cardioid patterns to reject onstage bleed. Drum overheads in cardioid minimize cymbal spill into tom mics. Broadcast hosts opt for cardioid lavaliers, rejecting ambient noise.
With good rear rejection and focused front pickup, cardioid mics deliver clarity for all kinds of miking needs. When directional isolation matters most, cardioids are probably the top choice. Their versatility makes them a modern workhorse.
Supercardioid Microphones
Supercardioid mics exhibit an even narrower pickup pattern than standard cardioids. Their highest sensitivity is dead ahead, with far less pickup off-axis and to the rear.
The supercardioid’s tight polar pattern provides excellent isolation and feedback rejection for live sound reinforcement, making it ideal for miking loud instruments.
They reject stage noise and bleed far better than cardioids. Rock vocalists often use supercardioid handhelds to cut through blasting bands.
However, supercardioids must be aimed very precisely at the sound source, making placement tricky. Any head movement can cause dramatic dropping out as talent moves off-axis.
In the studio, supercardioids excel as drum overheads, allowing loud cymbal hits without leakage into close mics on drums and hi-hats.
Their pronounced proximity effect gives supercardioids enhanced low-mid warmth and thickened vocals when close-miked. But off-axis highs can dull noticeably.
Though less forgiving of positioning than cardioids, supercardioids provide excellent isolation of loud sounds. Their tight polar pattern tames bleed and feedback.
Hypercardioid Microphones
The most directional cardioid variant, hypercardioid mics exhibit maximum front pickup with sizable rear rejection and serious off-axis attenuation.
Their tighter polar pattern improves isolation over even supercardioids. Live vocalists favor hypercardioids for cutting through rock and metal mixes with minimized bleed.
In the studio, hypercardioids minimize cymbal and hi-hat leakage when used as drum overheads. Their pronounced proximity boost warms close-miked instruments and voices.
However, hypercardioids are extraordinarily positioning-dependent. Even small movements off the central axis cause drastic drops in level. They require talent to remain still and steer the mic on axis.
For this reason, broadcast tv hosts tend to prefer standard supercardioids over hypercardioids for lavaliers. The latter leaves no room for head motion.
When extreme isolation is paramount, hypercardioids excel. Their pronounced proximity effect and narrow sensitivity window make close on-axis use optimal. Handle with care.
Bidirectional Microphones
Bidirectional or figure-8 microphones pick up sound equally from the front and rear, while rejecting sound from the sides.
Their polar pattern looks like a figure 8. Ribbon microphones commonly have bidirectional polar patterns, but other mic types can also be figure-8s.
In the studio, figure-8s are useful for stereo recording techniques like the Blumlein pair and mid-side miking. Their side rejection prevents phase issues.
For miking loud guitar amps or drum kits, bidirectional mics aimed forward can isolate better than cardioids, since the rear null avoids rear bleed.
In live use, rear rejection aids feedback control. Pointing the rear null at monitors reduces ringing. A hypercardioid can fill the rear null with reflected ambience.
However, bidirectional mics must be positioned symmetrically between front and rear sound sources to capture both effectively. This makes them inconvenient for handheld vocal use.
With strategically placed absorption at the rear, figure-8s can provide directionality rivaling cardioids for spot miking. Their strengths suit critical studio applications.
Other Polar Patterns
While omnidirectional, cardioid, supercardioid, and bidirectional patterns dominate microphone designs, other more specialty patterns exist:
- Subcardioid – A wide cardioid pattern with more rear pickup. Provides some ambient sound while still rejecting direct rear signals.
- Lobar – Unidirectional pattern with narrow front and rear sensitivity lobes. Requires precise positioning.
- Short shotgun – Extremely narrow polar pattern covering distance. Provides camera mic directionality.
- Wide-angle cardioid – Wider sensitivity than typical cardioid, approaching omnidirectional. Adds room ambience.
- Toroid – Doughnut-shaped pickup. Most sensitive at sides, rejects front/rear sound. Good for own voice pickup.
- Hexbidirectional – Figure-8 with added rear-facing lobe. Creates immersive spatial pickup.
- Variable-D – Continuously adjustable pattern. Allows tuning mic directivity.
While these exotic patterns fill specialty applications, the conventional polar patterns handle most needs.
But adjustable patterns allow room fine-tuning, and alternatives like toroid address specialized miking challenges. The quest for the perfect polar response continues.
Microphone Frequency Response
The frequency response of a microphone indicates how faithfully it reproduces the full audible frequency spectrum from the lowest bass tones to the highest treble.
Let’s explore what microphone frequency range means, how response varies between mic types, and why a balanced frequency reproduction is vital for professional audio work.
Microphone Frequency Range
The full spectrum of human hearing stretches from 20 Hz to 20 kHz. This means the ideal microphone would accurately and evenly reproduce this entire audible frequency range.
In reality, even exceptional mics have some deviation from totally flat response. But the very best aim for:
- Usable response down to 20 Hz to capture deep bass and sub-bass energy
- Smooth midrange response across the 100 Hz to 5 kHz vocal range without drastic spikes
- Extended high frequency response reaching 20 kHz to convey detail and “air”
However, most affordable mics are limited to 100 Hz to 15 kHz, rolling off the extreme lows and highs. Their tailored response targets the primary vocal range.
Dynamic vocal mics accentuate 2-5 kHz for clarity at the expense of weak bass and soft treble. Measurement mics may reach down to 10 Hz and up to 40 kHz for scientific analysis.
Any coloration should ideally complement the intended sound source. For example, a boosted 10 kHz range adds shimmer to cymbals.
The best studio mics capture 20-20,000 Hz smoothly. But real-world response compromises are inevitable. The art is balancing character and truth.
Microphone Frequency Charts
A microphone’s frequency response is commonly visualized in a chart with frequency on the X-axis and decibel level on the Y-axis.
The shape of this response curve reveals a mic’s particular coloration. Flatter is generally more neutral. Peaks and valleys alter the timbre.
For example, a vocal mic may show a pronounced 2-5 kHz boost for clarity and articulation. A ribbon mic will display an extended 10-20 kHz response lacking in typical dynamics.
Roll-off curves on both bass and treble extremes are common. These attenuate noise and avoid distortion from boosting extremes. Too much rolloff leaves a narrow, nasal midrange.
Ruler-flat response extending 20-20,000 Hz implies accurate translation but ignores noise. It’s rare except in the highest-end reference mics.
Consulting frequency charts helps match mics to sources. Analyzing the curves explains why a bright condenser suits acoustic guitar while a punchy dynamic fits a male rock vocalist.
Factors Affecting Microphone Frequency Response
A microphone’s frequency response depends on various design and usage factors:
- Capsule size – Larger diaphragms reproduce more low end. Small capsules lack bass.
- Diaphragm material – Lighter membranes boost high frequencies. Heavier ones favor low end.
- Vents/ports – Back vents extend highs. Front ports add lows. Sealed mics have reduced extremes.
- Proximity effect – Bass boost from close miking below 100 Hz. More pronounced in cardioids.
- Placement – Distance miking reduces bass. Nearby surfaces add reflections and comb filtering.
- Sound source – The frequency content being reproduced shapes the mic’s effective response.
- Polar pattern – Off-axis sounds are typically deficient in high frequencies.
- Accessories – Windscreens, grilles, shock mounts, and pop filters alter response.
Considering these many factors helps explain mic subtleties and match the right models to sources. Small changes make a big sonic difference.
Microphone Sensitivity
Microphone sensitivity indicates how well a microphone converts acoustic energy into electrical energy. More sensitive mics produce higher output for the same sound input.
Sensitivity is measured in mV/Pa, or decibels relative to 1 Volt/Pascal. Let’s look at how it’s quantified and which mic types have the greatest sensitivity.
Measuring Microphone Sensitivity
Sensitivity is measured in two main ways:
Millivolts per Pascal (mV/Pa)
This specifies the electrical output voltage from the mic in millivolts when subjected to a sound pressure of 1 Pa.
Typical sensitivities for small diaphragm condensers range from 10 mV/Pa to 50 mV/Pa. Dynamics have lower sensitivity, from around 1 mV/Pa to 10 mV/Pa.
Decibels relative to 1 Volt per Pascal (dBV/Pa)
This states the microphone’s sensitivity relative to a 1 V/Pa output across its frequency range. Condenser mics often range from -20 dBV/Pa to -40 dBV/Pa. Dynamics span -45 dBV/Pa to -55 dBV/Pa.
Higher dBV/Pa and mV/Pa figures indicate greater sensitivity. But ultra-high sensitivity can lead to overload distortion on loud transients.
Matching microphone sensitivity to the sound source level is crucial. High sensitivity condensers suit quiet acoustic instruments. Rugged dynamics work better for amplifiers and drums.
Microphone Sensitivity Ratings
Understanding published microphone sensitivity ratings helps match mics to intended sources. Here are typical sensitivity figures for common mic types:
- Small diaphragm condenser – -35 to -45 dBV/Pa, highly sensitive for acoustic instruments.
- Large diaphragm condenser – -28 to -38 dBV/Pa, ideal for studio vocals and piano.
- Dynamic vocal mic – -52 to -58 dBV/Pa, tailored for close speech pickup.
- Dynamic instrument mic – -45 to -55 dBV/Pa, handles loud guitar amps.
- Ribbon mic – -50 to -60 dBV/Pa, lower output but transparent sound.
Measurement mics used for acoustic analysis boast an extremely high sensitivity rating down to -26 dBV/Pa in order to capture room dynamics precisely.
These figures help explain why low sensitivity dynamic mics are preferred for miking loud sources like guitar amps and drums. Their robust coils handle intense SPLs without distortion.
Condensers better serve delicate acoustic instruments and nuanced vocalists. Their high sensitivity brings out subtle details.
So check mic specs and match sensitivity to the sound source. This optimizes level, headroom, and signal quality.
Microphone Self-Noise
Microphone self-noise refers to the inherent noise floor present when a mic isn’t picking up any sound. This noise stems from electronic and mechanical factors within the mic itself.
The lower the self-noise, the better the signal to noise ratio. Let’s examine the causes of self-noise and how microphone design affects it.
Causes of Microphone Self-Noise
Even when no sound is entering a microphone, its output contains a faint hiss or hum. This inherent noise floor stems from several internal sources:
- Electronic noise – The mic’s internal amplifier circuits produce thermal and current noise from components like resistors and transistors. More complex circuitry increases noise.
- Mechanical noise – Physical vibrations and friction between moving parts like ribbon coils and dynamic diaphragms create low level noise. Internal shock mounting helps reduce it.
- Power supply noise – External phantom power or internal battery ripple and fluctuations can modulate the audio signal. Clean steady power is ideal.
- Thermal noise – Due to the random motion of electrons in mic wires and components, even resistors produce noise dependent on their values.
Condenser mics tend to have lower noise since they lack moving parts and have simple internal amplifier circuits. Transformerless FET designs further remove noise sources.
Choosing low-noise mics and providing clean stable phantom power prevents amplification of unwanted background. This maximizes the microphone’s useful signal to noise ratio.
Minimizing Microphone Self-Noise
While some background hiss is inherent to any mic, there are ways to reduce self-noise and improve signal quality:
- Choose low-noise mics, especially for quiet or acoustic sources. Condensers tend to be lower noise than dynamics.
- Use balanced XLR cables to reject electromagnetic interference. Avoid long unbalanced lines.
- Phantom power supplies should be clean and ripple-free. Use batteries if plug-in power is noisy.
- For ribbon and dynamic mics, use preamps with high input impedance as this reduces thermal noise.
- Shield cables from electromagnetic fields caused by lighting, power lines, computers, and other electronics.
- Aim for high signal levels without overload – this maximizes the signal to noise ratio and masks hiss.
- Use noise gating either during recording or in post to mute noise during pauses. But avoid heavy gating that removes room ambience.
With quality modern mics and proper gain staging technique, self-noise should remain at very low background levels compared to the desired signal. A bit of hiss need not harm most recordings.
Microphone Power Requirements
Microphones need electrical power to run their internal circuitry and componentry. Let’s examine common ways to power different mic types.
Phantom Power
Phantom power is the standard method of energizing condenser microphones. It refers to DC voltage carried on the same wires as the audio signal in a balanced XLR cable.
Typical phantom power supplies provide 48V DC at low current to drive the amplifier and electronics built into condenser mics. Dynamic mics can usually tolerate phantom power through blocking capacitors.
Batteries
Some microphones rely on internal batteries to provide power rather than external phantom power. These often include wireless lavalier mics and consumer device mics.
Alkaline and lithium batteries are common. Rechargeable designs offer the convenience of avoiding battery swaps. Battery life varies widely between models and brands.
Other Options
Ribbon and dynamic mics are passive and require no power. But some preamps for these mics offer 12-24V “phantom power” simply to feed LED indicators.
Alternatives like USB or dedicated proprietary connections can power mics like headsets and phone accessories.
Next let’s explore which mic types utilize these different powering methods and why.
Microphone Applications
One of the keys to choosing the right microphone is understanding the wide variety of applications and uses for different models. Let’s explore some of the top microphone applications.
Studio Recording
The versatile condenser microphone reigns supreme in professional recording studios. Their high sensitivity, extended frequency response, and excellent transient response capture intricate details critical for modern multi-tracked production.
Large diaphragm condensers owe their popularity to silky, robust low end and crisp defined highs that flatter vocals and acoustic instruments. Their wide sweet spot tolerates more distant miking.
Small diaphragm condensers lend sparkling presence to drum overheads, acoustic guitars, percussion, and backing vocals. Their precision suits pristine choral recording.
Ribbon microphones add vintage warmth to electric guitars, brass, woodwinds, and room ambience. Their smoothness tames harsh digital edges.
Robust dynamic microphones reliably track bass amps and loud drum kits. Their selective midrange punches through the mix.
Careful studio miking requires using the right mic in the right spot. Blending multi-mic elements gives control over each aspect of the sound during mixing.
This creative flexibility to sculpt sound explains why condensers dominate studio use. Their purity provides a canvas for molding polished professional recordings.
Live Sound Reinforcement
Miking live performances poses distinct challenges that influence microphone selection. Sound engineers mixing concerts, theater, conferences, and other events need mics tailored to these demands.
Vocalists require handheld dynamic microphones for their natural presence boosting articulation and feedback resistance for monitors.
Headset condensers free actors and singers to dance and move without microphone handling noise.
Drum microphones are predominantly robust dynamics that withstand blistering loud volumes at close proximity. Overhead condensers add cymbal sheen.
DI boxes convert signals from instruments like electric guitars to balanced XLR for long mic cable runs. Acoustic instruments use condenser mics.
Lectern microphones are often mounted goosenecks allowing adjustable positioning. Lavaliers work for television and theater.
Feedback control is essential. Directional polar patterns, carefully aimed stage monitors, and judicious use of parametric EQ are key tools.
Reliability and durability matter onstage. Live sound mics must render crisp amplified sound night after night.
Broadcast
Microphones are crucial for television, radio, podcasts, and other broadcast media. They offer listeners an intimate lifeline to remote hosts.
For interviews, handheld cardioid dynamics allow guests freedom of movement and proximity control while rejecting off-axis noise.
Hosts rely on lavalier condensers, often mounted in lightweight headworn arrays for complete mobility. Their tighter pickup avoids plosives.
In-studio broadcast boom mics use interchangeable supercardioid capsules for directional talk pickup. Permanent studio condensers capture room ambience.
Field reporters need highly directional shotgun microphones to isolate dialogue from busy crowds and locations. Windscreens and shock mounts reduce vibration and rustling.
Wireless ENG camera crews use compact plug-on transmitters to convert their shotgun mics for cable-free use over distances.
For sports, specialized mics withstand weather while amplifying calls on the field. Studio analysts get private headset microphones.
Delivering articulate voices and soundscapes without distortion or distraction is thebroadcast microphone’s purpose. Their sound transports audiences to events both global and local.
Measurement
Precision measurement microphones provide accurate acoustic analysis for scientific research and industrial development.
Typically small condenser microphones coupled to a 1/4″ or 1/2″ pressure-field prepolarized capsule, these reference mics have an exceptionally flat frequency response to 150 kHz with under 15dBSPL noise floors.
Extremely low distortion allows capturing the nuances of spatial phenomena like speaker cone excursion under high power. Deviation less than 0.5 dB across an enormous 140 dB range qualifies reference mics.
Careful orientation and positioning, often using calibrated laboratory-grade stands, ensures meaningful quantitative results. Windscreens prevent turbulence.
Applications range from examining noise in jet engines and rocket launches to studying auditorium acoustics, loudspeaker parameters, and hearing aid performance.
For analyzing our dynamic soundscape with scientific rigor, reference measurement microphones grant insightful precision unattainable otherwise. Their pristine translation expands acoustic knowledge.
Miking Instruments and Voice
Certain microphone models excel at capturing specific sound sources and scenarios:
- Vocals – Large diaphragm condenser or dynamic handheld mics warmly flatter the human voice. Proximity effect adds fullness.
- Acoustic guitar – Small diaphragm condensers bring out genteel strings and fretwork while avoiding boominess.
- Electric guitar amp – Robust dynamic mics take crunching decibels in stride. Ribbons smooth harsh edges.
- Piano – Large diaphragm condensers capture a balanced stereo image. Close miking adds percussiveness.
- Drum kits – A blend of dynamics for kick and toms and condensers for cymbal clarity and roomy overhead miking.
- Orchestras – Spaced omnidirectional condensers convey a lush stereo ambience. Spot mics reinforce solos.
- Percussion – Condensers catch crisp highs and realistic room reflections. Proximity effect warms bass drums.
- Public speaking – Cardioid dynamics resist feedback while projecting authoritative voices clearly.
- Acoustic bass – Pickup the deep lows with a large diaphragm dynamic or condenser, sometimes using both a direct mic and a bass amp mic.
- Brass instruments – Condensers add sheen and preamp warmth helps smooth brass. Ribbons tame blaring trumpets and trombones.
- Woodwinds – Small diaphragm condensers capture subtle intonations without distorting.
- Choir – Spaced small or large diaphragm condensers blend voices in cathedrals. Cardioids up close isolate sections.
- Conference calls – Lavalier mics allow hands-free communication. Noise-canceling models reduce ambient noise.
- Field interviews – Shotgun mics isolate voices from a distance while on-camera condenser lavaliers pick up clean speech.
- Voice over – Large diaphragm condensers flatter baritones while reducing pops. Studio deadening prevents reverb.
- Podcasting – USB condensers offer simplified connectivity and sound for amateur recording.
- Gaming – Headset mics with background noise reduction aid online communication. Omnidirectional pickup adds immersion.
- Mobile use – Miniature omni electret condenser capsules built into phones and tablets allow call clarity.
Considering the tonal balance, dynamics, and pickup patterns best suited to the sound source leads to matching the ideal microphone models for vibrant musicality and articulate speech.
Microphone Specifications
Various technical specifications help determine which microphone is best suited for a particular application. Let’s explore some of the key parameters.
Impedance
A microphone’s electrical impedance, measured in ohms, indicates how much resistance it presents to AC current flow. Typical microphone impedance ranges from 150-600 ohms.
Low-impedance microphones around 150-250 ohms work well with long cable runs because the low resistance minimizes high frequency signal loss over distance. However, low-impedance mics are more prone to interference.
High-impedance microphones around 600 ohms exhibit higher output impedance that reduces induced noise in the microphone signal itself. But long unbalanced cables with a high-impedance mic source can degrade high frequency response.
Dynamic microphones tend toward lower impedance while condenser and ribbon microphones typically have higher impedance outputs.
Proper preamplifier impedance matching minimizes frequency response alterations. For example, a 10,000 ohm input impedance or higher is recommended for 600 ohm ribbon mics. Low-impedance mics can tolerate much lower inputs.
Considering microphone impedance along with required cable lengths helps maintain smooth frequency response from the mic itself all the way to the preamplifier or mixer input.
Maximum SPL
A microphone’s maximum SPL indicates the highest sound pressure level it can handle before distorting. This spec helps determine if a mic is suited to loud or quiet sources.
Condenser microphones typically max out at 130 to 140 dB SPL, sometimes as low as 120 dB for small diaphragm models. Their high sensitivity makes condensers prone to overloading.
Dynamic microphones excel at high volumes, with rugged moving coil models handling 160 dB SPL or even higher. This makes them suitable for close miking loud guitar amps and drum kits.
Ribbon microphones are the most fragile, with max SPL around 135 dB. Sound levels beyond this can easily damage the delicate ribbon element.
For loud rock vocals and instruments, robust dynamics handle intense SPLs. But for quiet acoustic instruments and nuanced singing, a condenser’s low noise and headroom is ample.
Considering the volume levels involved guards against distortion while allowing sufficient signal level into the preamp or mixer for proper gain staging.
Signal-to-Noise Ratio
A microphone’s signal-to-noise (S/N) ratio compares the level of the desired signal to the level of background noise when no intentional sound is entering the microphone.
Higher S/N ratios indicate less self-noise and better sound quality. Dynamic mics often have S/N around 60-70 dB, while large diaphragm condensers boast 80 dB or better.
However, S/N ratios above 70-80 dB make little perceptible difference, as room noise and preamp noise mask mic self-noise. Extremely high S/N only helps scientific measurement.
When recording loud signals like vocals, drums, and guitars, moderate S/N ratios around 60-70 dB suffice. But quiet acoustic instruments benefit from condensers with S/N of 80 dB and up.
Considering self-noise in conjunction with the mic’s sensitivity rating gives a complete picture. Lower noise mics prevent hiss while higher sensitivity extracts detail. But ultra-low noise won’t help noisy louder sources.
All the Important Specifications and What They Mean
Understanding microphone technical specifications demystifies choosing the right models for particular needs. Here are some top specs and what they indicate:
- Frequency Response – The mic’s reproduction of bass, mids, and treble. Wider and flatter is more neutral.
- Polar Pattern – The mic’s sensitivity to sounds arriving from different angles. Patterns improve isolation.
- Sensitivity – How well the mic converts acoustic energy to electrical signals. Higher sensitivity means more output level.
- Impedance – The mic’s electrical resistance. This affects cabling needs and preamp matching.
- Max SPL – The highest sound pressure level handled before distorting. Important for loud sources.
- S/N Ratio – Compares signal to noise floor. Higher is better to reduce hiss. But >70 dB is often adequate.
- Power Requirements – Whether the mic needs phantom power, batteries, or no power.
- Equivalent Noise – The mic’s noise level expressed as an electrical signal. Lower numbers mean less self-noise.
- Maximum Output – The highest output voltage the mic can produce before clipping. Allows matching levels.
- Capsule Size – The diaphragm dimensions. Generally larger diaphragms have better low frequency response.
- Weight – Important for handheld and headworn mics. Lighter models allow easier use.
- Dynamic Range – The decibel levels between lowest noise and maximum SPL. Wider is better.
- Total Harmonic Distortion – The mic’s alteration of the sound waveform. Lower distortion is more accurate.
- Off-Axis Rejection – Attenuation of sounds arriving from the side. Improves isolation.
- Proximity Effect – Boosting of bass frequencies when used up close. Impactful on some vocals.
Analyzing specifications reveals the mic’s ideal applications. A flat wide-ranging condenser suits pristine acoustic recording. A rugged high SPL dynamic excels on the drum kit. Specs quantify performance.
Microphone Connectors
Microphones use a variety of plugs and jacks to connect to other equipment. Some common types include:
XLR Microphone Connectors
The XLR connector is the standard for balanced professional microphone cabling. XLR plugs and jacks are found on most professional mics, cables, mixers and preamplifiers.
XLR connectors provide:
- Balanced signal transmission to reduce noise in long cable runs
- Secure locking mechanism preventing accidental disconnection
- Rugged construction for heavy use and repeated mating cycles
- Three conductors (ground, +signal, -signal) for stereo compatibility
- Established standard ensures universal compatibility between microphone and device connections
XLR wiring:
- Pin 1: Ground
- Pin 2: Positive signal (hot)
- Pin 3: Negative signal (cold)
Gold-plated contacts prevent corrosion for reliable connections. Neutrik, Switchcraft, Amphenol and Neutric are leading XLR connector manufacturers.
For critical connections, XLR is the tried and trusted choice of audio professionals. Its balanced design and robust build make XLR the industrystandard for microphone cabling.
USB Microphone Connectors
USB (Universal Serial Bus) has become a common standard for connecting microphones directly to computers, mobile devices, and audio interfaces.
Benefits of USB microphones:
- Simple plug-and-play connectivity – no separate interface needed
- Portable convenience for laptop and mobile recording
- Enables basic quality recording with affordable mics
- Built-in ADC conversion from analog to digital
- Bus-powered operation requires no external power supply
- Driverless operation with standard USB audio class support
Limitations include:
- Cable length limits of around 10-15 feet
- Potential issues from noisy computer power
- Limited compatibility with professional balanced audio gear
For basic recording needs, USB microphones provide a convenient and affordable option. But for mission critical studio work, XLR mics fed into external preamps and interfaces deliver superior sound quality and connectivity.
TRS Microphone Connectors
The 1/4″ TRS (Tip Ring Sleeve) connector is commonly used for unbalanced microphone connections.
TRS provides:
- Ubiquitous compatibility with mixers, guitars, and consumer devices
- Longer cable runs than XLR due to unbalanced design
- Two conductor connections (tip and ring) for simple stereo
- No locking mechanism, connections can vibrate loose
- Mono TS (Tip Sleeve) connectors also compatible
TRS wiring:
- Tip: Positive signal
- Ring: Negative signal
- Sleeve: Ground
TRS is more prone to interference than balanced XLR over long distances. Converters like the direct box allow connecting unbalanced mics to balanced XLR inputs.
The standard 1/4″ jack makes TRS a convenient option for instruments, headphones, desktop mics and other applications where balanced XLR is unnecessary.
Mini-Jack Microphone Connectors
Mini-jacks refer to the common 2.5mm and 3.5mm TRS connectors used in compact consumer microphone products.
Benefits of mini-jacks:
- Compact size suits lavalier mics, headsets, smartphones
- Ubiquitous compatibility with mobile devices and laptops
- Allows extended length unbalanced connections
- Often used for basic mono microphone signals
Downsides of mini-jacks:
- No standard for TRS stereo wiring
- Limited ruggedness for heavy use compared to XLR
- Unbalanced design picks up interference
- Not a common professional audio connector
While XLR and 1/4″ TRS connections dominate pro gear, mini-jacks are the standard for affordable consumer mics and mobile accessories. They excel in portable applications prioritizing compactness over fidelity.
Other Microphone Connectors
Beyond XLR, USB, and 1/4″ TRS, some less frequently used connectors also serve niche microphone needs:
- Proprietary Connectors: Many headset, lavalier, and wireless microphone systems employ custom connectors optimized for their specialized needs. Benefits include robustness, compactness, and dedicated extra connections. Drawbacks are lack of compatibility with XLR gear and cables.
- DIN Connectors: Early synthesizers and home computers sometimes utilized DIN connectors for peripherals including microphones. They provide more pins than XLR or TRS but aren’t as rugged.
- Modular Connectors: Modular plugs with 4-6 pins were popular in telephony and radio broadcasting for patch bays and multi-line connections. They enabled tapping signals between sources.
- BNC Connectors: The bayonet locking BNC connection used primarily in RF and video applications occasionally serves measurement mics. It enables quick connection/disconnection.
- Terminal Block Connectors: Screw, spring, and lever terminal blocks allow daisy chaining multiple low impedance mics for paging and surveillance systems.
While XLR has emerged as today’s pro standard, exploring options like mini-jacks or proprietary plugs can serve unique portable microphone needs.
Microphone Placement Techniques
Proper microphone positioning is crucial for achieving quality recordings and minimizing issues. Placement impacts tone, isolation, and other factors. Let’s explore some key microphone placement considerations.
Mitigating Proximity Effect
Proximity effect refers to the increased bass response that results when directional microphones are placed very close to sound sources.
This effect is most pronounced in cardioid mics when used within 2-3 inches of vocals and instruments. It can provide pleasing warmth but also risks muddiness.
To control proximity effect:
- Find the “sweet spot” distance with well-balanced tone, usually 4-12 inches for vocals.
- Use omnidirectional mics to avoid proximity effect, or cardioids in omnidirectional mode.
- Angle downward-facing mics so sound strikes diaphragm obliquely, reducing bass emphasis.
- Employ high-pass filters or EQ cut to reduce excessive “booming” bass buildup.
- Ribbon and condenser mics exhibit less pronounced proximity boost compared to dynamics.
Proximity effect is a tool to exploit deliberately for enhanced lows. But minding distance helps prevent a microphone’s natural bass emphasis from becoming overbearing.
Plosive Protection Techniques
Plosives are the distinctive “popping” sounds produced by vocalists on hard consonants like P, T, K, B, D, and G. Without plosive protection, these gusts of air can distort mics.
To prevent plosives:
- Use a windscreen, pop filter, or mic grill to diffuse blasts before they strike the diaphragm.
- Angle the mic 10-45 degrees off-axis so plosives glance the diaphragm rather than impact it head-on.
- Position the mic just off the side of the mouth rather than directly in front.
- Place the mic below the mouth, so plosives dissipate before reaching the capsule.
- Employ a shock mount to isolate the mic if plosives still cause thumps.
With proper positioning and diffusion, plosives can be tamed. This allows close-miking vocals without distracting blasts compromising recordings. A little prevention goes a long way.
Microphone Placement for Stereo Recording
Capturing a realistic stereo image involves thoughtful microphone placement. Here are some top techniques:
Spaced Omnidirectional Mics
Placing two omnis several feet apart captures a natural room ambience. Their distance creates intensity and timing differences between left/right channels.
Coincident Pairs
Two directional mics right next to each other maintain acoustic perspective. Popular coincident techniques include XY (angled 90-110 degrees) and Mid-Side.
Near-Coincident Pairs
Slightly spaced directional mics enhance width and channel separation. The ORTF and NOS stereo techniques use small angled spacing.
Decca Tree
This classically-used studio ensemble has 3 omni mics spread in a wide tree arrangement. It provides a full “3D” soundfield.
Multichannel
Additional mics like outriggers, room mics, and spot mics build an immersive soundscape. 5.1 surround recording expands the stereo field.
Meticulous microphone placement is key to capturing a believable, pleasing stereo image with good blend, perspective, and spatial realism.
Optimizing Room Acoustics
The sound of any recording space profoundly influences microphone pickup. Wise placement minimizes problems from room acoustics.
For vocal clarity:
- Place mics in carpeted/furnished areas absorbing reflections
- Angle mics to limit pickup of problem surfaces like glass or tile
- strategic acoustic treatment prevents flutter echo and ringing
For natural ambience:
- Distance omni mics 3+ feet from sound sources
- Lift mics overhead into a diffuse sound field
- Place room mics widely for realistic reverb balance
For separation:
- Keep directional mics close to reject room sound
- Spot mics target direct sound avoiding room bleed
- Physical sound barriers and baffling prevent crosstalk
Understanding a room’s acoustic qualities allows optimizing placement for clarity, warmth, and separation. Tame the space, don’t let it tame your sound.
Microphone Accessories
Supporting gear like cables, stands, and mounts optimize microphone performance. Here are some essential add-ons.
Cables
Quality XLR, TRS, and USB cables ensure clean signal transfer and durability. Keep runs short or use balanced connections.
Stands
Floor, desk, and boom stands position mics precisely. Flexible goosenecks aid adjustment. Shock-absorbing bases reduce vibration.
Shock Mounts
Elastic suspension isolates mics from stand/mount vibrations and rumble. Useful for mics prone to handling noise.
Pop Filters
Mesh screens diffuse plosives and breath blasts before they distort mics. Vital for close-miked vocals.
Windscreens
Foam or fur hoods prevent noise from wind and ventilation air. Essential for location filming.
Preamps
Dedicated preamps and DI boxes boost and condition mic signals before the mixer. May add warmth.
Quality supporting gear maximizes recording and performance results. The right accessories safeguard microphone integrity.
The Future of Microphones
Microphones have come an enormous way since their crude beginnings. But many thrilling innovations still lie ahead. Let’s explore what the future may hold.
Cutting-Edge Microphone Innovations
Exciting advances in materials science and electronics promise to reshape microphone design.
MEMS Microphones
Micro-electro-mechanical systems shrink entire mic components onto silicon chips. MEMS mics enhance mobile devices and hearing aids through extreme miniaturization.
Metamaterials
Engineered composites exhibit extraordinary properties like perfect sound absorption. Metamaterial coatings can eliminate ambient noise pickup in mics.
Graphene Diaphragms
Graphene’s high stiffness and low mass can enable more responsive condenser mic diaphragms. Some prototypes already exploit graphene.
Quantum Microphones
Quantum tunnel junctions convert sound waves into electrical signals through quantum effects, enabling extreme sensitivity.
Optical Microphones
Fiber optic hydrophones sense minute pressure variations underwater or in other harsh environments using laser interferometry.
Cutting-edge materials and nanofabrication push performance limits ever further. Where microphone innovation will ultimately lead is still unfolding.
Microphones for 3D and Spatial Audio
Next-generation immersive audio relies on advanced microphone techniques to capture spatial realism.
Spherical Arrays
Circular multi-mic rigs record in all directions, placing listeners at the center of an ambient soundfield.
Binaural Dummy Heads
Special mics mounted in a model human head mimic actual ear positioning for life-like 3D sound.
Ambisonics
Using multiple capsules, ambisonics mics encode directional sound data for decoding over surround speaker setups.
Object-Based Audio
Object metadata captured by advanced mics allows precise positioning of sounds in 3D space.
VR/AR Recording
Spatial mics tailored for VR and AR capture environmental acoustics and positional cues for heightened realism.
Immersive audio relies on mics providing complete spherical coverage and spatial cues hinting at direction and distance. The tech aims to put you right inside the scene.
AI and Machine Learning Microphone Advances
Intelligent algorithms are unlocking new microphone capabilities.
Noise Cancellation
AI isolation separates voices from background noise in real time using layered neural networks.
Source Separation
Algorithms extract individual sounds from complex microphone arrays, isolating voices, instruments, and effects.
Auto Mixing
Machine learning models dynamically adjust channel levels, EQ, and compression for optimal balance and clarity.
Metadata Generation
AI can automatically tag and index vocalists, venues, moods, and objects throughout recordings.
Voice Control
On-device voice assistants use mics with noise cancellation and contextual understanding to handle commands.
Sophisticated artificial intelligence grants microphones powers of discernment and perception surpassing human hearing. The future of how mics understand our world is near.
Final Thoughts
From the earliest telephone voice transmission to state-of-the-art surround sound, the microphone has completely transformed how we capture and connect with the world around us.
What began as a simple method of converting acoustic waves into electrical signals has grown into an incredibly diverse realm of ultra-precise tools tailored to countless applications.
Yet at their core, even the most advanced microphones remain based on those fundamental electromagnetic principles uncovered over a century ago. The genius of pioneers like Emile Berliner, Alexander Graham Bell, and James West gave birth to the microphones we rely on today.
Now with emerging frontiers in fields like MEMS, AI, and 3D audio, the microphone’s future promises to be even more incredible than its past. Wherever these technologies take us, the ability to transduce sound with elegance and sensitivity will remain crucial.
From vintage ribbon mics to subminiature lavs, these clever inventions empower us to share ideas, connect emotionally, and experience the world together. The microphone amplifies the resonances of our shared humanity.
So the next time you make a call, listen to music, or record your voice, pause to appreciate the extraordinary microphones making it possible. They truly are marvels of engineering in miniature. The sound of progress starts here.
Frequently Asked Questions
Microphones play a vital role in many aspects of our lives, from personal communication to art and entertainment. But how exactly do they work, and what should you consider when choosing one? Here are answers to some common microphone FAQs.
What’s the main difference between dynamic and condenser microphones?
The primary distinction is how they convert sound into electrical signals. Dynamics use electromagnetic induction – sound vibrates a coil in a magnetic field, generating a current. Condensers have an electrically charged diaphragm that forms a capacitor with the backplate. Sound vibrations alter capacitance.
Condensers offer extended frequency response and higher sensitivity but require external power. Dynamics handle high volumes without distortion.
What polar patterns are best for isolation versus natural reproduction?
Cardioid, supercardioid, and hypercardioid patterns provide isolation by rejecting off-axis sounds. This focus makes them ideal for spot miking instruments and vocalists.
Omnidirectional mics pick up sound evenly from all directions. This provides a natural reverberant sound but lacks noise rejection. Great for room miking.
When would you choose a ribbon mic versus a condenser or dynamic?
Ribbon mics excel at smooth, detailed reproduction of high frequencies from cymbals to brass. Their figure-8 polar pattern also helps control room reflections.
However, ribbons are more fragile and lower output than condensers and dynamics. Use ribbons when a natural, vintage-flavored sound is preferred.
What accessories are must-haves versus just nice-to-haves?
Pop filters and windscreens are essential for quality vocal recording. Shock mounts safeguard expensive condensers. Quality cables maintain signal integrity.
Preamps, tube mic emulations, and advanced stands offer benefits but aren’t mandatory. Prioritize necessities over frills.
What should you avoid to extend microphone life?
Prevent moisture exposure or damage from plosive blasts. Store mics properly. Avoid dropping or excess vibration. Maintain cables gently. For condensers, keep capsules clean and have them calibrated periodically. With care, mics can last decades.
Why do microphones need phantom power?
Condenser mics require phantom power – 15-48V DC on the audio cable – to charge their capacitive transducer. This power energizes their internal amplifier circuitry.
Dynamics don’t need phantom power.
Why use ribbon mics on guitar amps instead of condensers?
Unlike condensers, rugged ribbon mics handle the intense sound pressure levels of loud guitar amps. Their smooth frequency response tames harsh midrange and adds pleasing warmth.
How can mic placement minimize phase cancellation issues?
Keep multiple mics equidistant from sources to avoid comb filtering from delayed reflections. Align cardioid off-axis nulls to reject problematic reflections. Minimize spill between open mics.